By Morgan Ahn
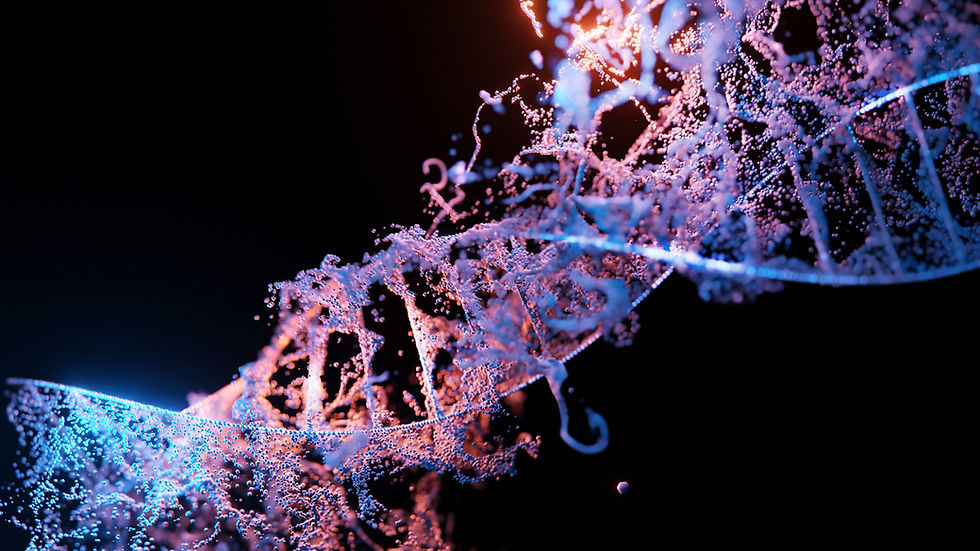
Introduction
“CRISPR is an acronym for Clustered Regularly Interspaced Short Palindromic Repeat which is a group of DNA (Deoxyribonucleic Acid) found in genomes of organisms such as bacteria.”
CRISPR-Cas9, often referred to as genetic scissors, is fascinating to me because it is a significant scientific breakthrough so far in the 21st century. Science magazine announced CRISPR-Cas9 genetic scissors as top 10 innovative technology last 2015: pulling down the limitation for medical treatment. Therefore, I wanted to research not only the ambiguity of this technology but also how it can be applied in a genuine situation.
Overview
Genetic scissors have been a keen interest for scientists – especially medical scientists since it greatly impacts the medical field. This research started in 1985 when Miller discovered the Zinc Finger. He began studying at the TFIIA in 1982 which led him to discover the method to grasp the DNA by binding specific DNA sequences with Zn. After a remarkable discovery, TALEN (Transcription activator-like effector nuclease) was discovered in 2010 by Dan Voytas. This was easier to use than the other technologies and opened the possibility of genome editing. Hence, the research in medicine rapidly progressed, and finally, in 2012, Jennifer Doudna and Emmanuelle Charpentier discovered CRISPR-Cas9. So, what is CRISPR-Cas9, and why do scientists break through the problems?
Structure
CRISPR-Cas9 is able to edit the genome by cutting a specific section of the DNA sequence or rectifying it. However, there are some differences compared to the previous technology. It does not have a complex protein structure and is capable of cutting DNA deeper and more precisely.
According to The Federal, CRISPR includes a guide RNA (gRNA) which is a “piece of pre-designed RNA sequence located within a longer RNA scaffold.” This can change the genomic target of the Cas enzyme by simply changing its sequence; and, it helps Cas9, an enzyme, to cut the properly planned part of the DNA – prevent cutting off-target – because gRNA has RNA that supports the target DNA sequence.
Occasionally, if gRNA were replaced with other RNA, it can be used as the genetic scissors that can cure other genetic diseases. Scientists were able to discover Cas9 by certain bacteria which have a similar gene-editing system as much as responding to pathogens. By elaborating on the technology, scientists say it is able to mutate DNA.
Function
The mechanism of the CRISPR-Cas9 genome editing has “three [main] steps: recognition, cleavage, and repair.” (The big bang of genome editing technology: development and application of the CRISPR/CAS9 system in disease animal models) Cas9 protein, which contains six domains – REC I, REC II, Bridge Helix, PAM Interacting, HNH, and RuvC – bind with gRNA and scan DNA sequences to find the particular sequence which is two G bases next to each other. If it finds such a sequence, Cas9 protein extends the double helix to check if it is complementary with gRNA. Under this condition, Cas9 cuts the strands of the DNA. After Cas9 is activated, the cell tries to minimize the damage by sticking ends together and mutating the end side of the break or searching the matching sequences to repair the certain deserted location. By the process of finding alternations of the particular sequence, scientists make it possible to set the genome as they desired.
There are various advantages that CRISPR-Cas9 brings to us. It can be the “potential tool” for the treatment of diseases such as cancer, hepatitis B, AIDS, and high cholesterol. Thus, a variety of people in different groups can use this method since it reduces time compared to the gene targeting technologies by directly applying it to the embryo, and gene editing by applying this technology is relatively inexpensive. Due to these conveniences, in 2021, according to the Innovative Genomic Institute, CRISPR technology is used to produce fetal hemoglobin – not curing the mutation – for treating blood disorders: Sickle Cell Disease (SCD) and Beta-Thalassemia. Scientists gathered the patients’ blood stem cells and edited their genomes. Then, abnormal blood cells – sickle cells – are killed by the chemotherapy and edited stem cells are injected into the bloodstream. As it circulates, it settles on the bone marrow and creates a new bloodstream – producing fetal hemoglobin. However, alongside the upper hand, since there is a high possibility to mutate the embryo as they want, ethical problems have been raised. In fact, in 2015, it was a cause celebre that one laboratory in China mentioned the success of embryo genome mutation in a medical journal, Protein&Cell. They mentioned that even though they used 86 non-viable embryos and attempted to edit the genome which causes fatal blood disease, only some of them were successful due to off-target mutation. Also, CRISPR-Cas9 can affect off-target cells and alter the function of the gene which might cause genomic instability and hinder clinical procedures.
Therefore, we cannot conclude that CRISPR-Cas9 is totally beneficial because several drawbacks and side effects exist.
Application/Conclusion
Genetic editing technology is rapidly changing. CRISPR-Cas9 was discovered only a few years after the discovery of TALEN. Besides, now, some have discovered the fourth generation of genetic scissors, Base editing, which is based on the CRISPR-Cas9 technology. It has a unique feature: editing the gene, but not cutting the helix. It is true that a lot more opportunities are available as we enhance technology, but we also need to assure the double-sidedness of it.
References
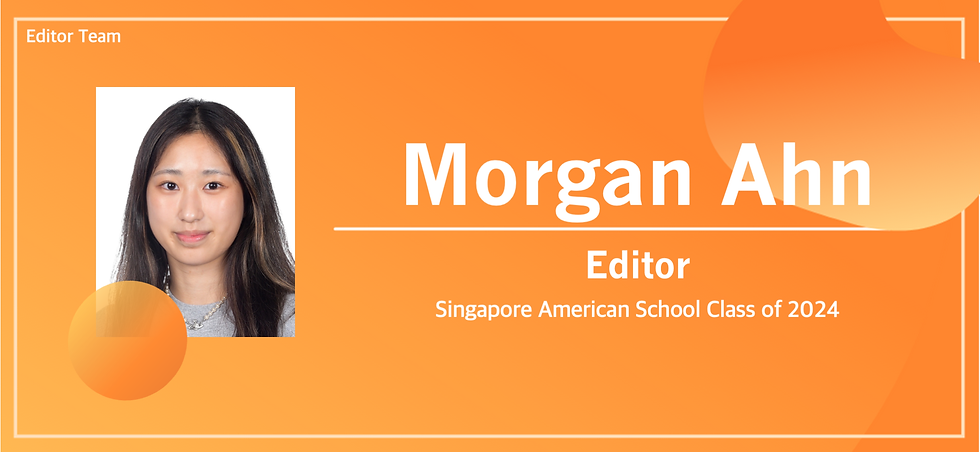
Comments